Magnetoencephalography in pediatric epilepsy
Article information
Abstract
Magnetoencephalography (MEG) records the magnetic field generated by electrical activity of cortical neurons. The signal is not distorted or attenuated, and it is contactless recording that can be performed comfortably even for longer than an hour. It has excellent and decent temporal resolution, especially when it is combined with the patient's own brain magnetic resonance imaging (magnetic source imaging). Data of MEG and electroencephalography are not mutually exclusive and it is recorded simultaneously and interpreted together. MEG has been shown to be useful in detecting the irritative zone in both lesional and nonlesional epilepsy surgery. It has provided valuable and additive information regarding the lesion that should be resected in epilepsy surgery. Better outcomes in epilepsy surgery were related to the localization of the irritative zone with MEG. The value of MEG in epilepsy surgery is recruiting more patients to epilepsy surgery and providing critical information for surgical planning. MEG cortical mapping is helpful in younger pediatric patients, especially when the epileptogenic zone is close to the eloquent cortex. MEG is also used in both basic and clinical research of epilepsy other than surgery. MEG is a valuable diagnostic modality for diagnosis and treatment, as well as research in epilepsy.
Introduction
Electrophysiologic evaluation is essential and critical for both clinical practice and basic research of epilepsy. Electroencephalography (EEG), which measures the voltage difference of brain electrical activities from the scalp, has played a critical role for more than half a century. Magnetoencephalography (MEG) is a relatively new technology that records the magnetic fields of the brain. Technological advancements had enabled researchers to detect the tiny magnetic field from the body. It is a completely noninvasive method that is used to evaluate normal and abnormal cerebral activities. It is a very precise method that has excellent spatial resolution of millimeters and temporal resolution of milliseconds. Widely accepted indications of MEG for pediatric patients are detecting spontaneous epileptiform activities and mapping eloquent cortices of motor, sensory, and language. Application of MEG in epilepsy has increased tremendously and also the number of research publications. It is considered as a useful evaluation modality in presurgical evaluation, intractable epilepsy, and cortical mapping. In this review, we will briefly introduce MEG and review the latest application of MEG in pediatric epilepsy.
Fundamentals of MEG
1. Brain magnetic field
Cellular currents from active neuronal population produce both electrical potential distribution on the scalp and magnetic field around the head. While EEG measures this potential difference from the scalp using directly contacted electrodes, MEG measures the magnetic field generated by the primary currents according to the "Right-hand rule of Ampere" by the completely contactless special sensors. Continuous recording of the changes in this magnetic field flux is called magnetoencephalogram. So both EEG and MEG measure the same electrical phenomenon in two different ways.
2. Superconducting quantum interference device sensors and shielding
Because the magnetic field generated by the brain is extremely weak (about one billionth of earth magnetic field, usually 50 to 200 femto-tesla), very sensitive sensors are needed to detect the changes of this tiny magnetic field. Superconducting quantum interference device (SQUID) sensors1) solved this problem, which is a special sensor that functions at a temperature of 4 K (-269℃) that detects the change of magnetic field flux over time2,3). These sensors are embedded in a helmet-shaped device called "dewar" which is filled with liquid helium to keep the temperature at 4 K. A magnetically shielded room is needed to block the preexisting environmental magnetic fields of cars, electricity and earth magnetism.
3. Examination procedure and interpretation of data
MEG machines that are mostly used worldwide are capable of performing the examination with the patient either lying down or sitting in the chair (Fig. 1). So we can easily perform the mapping of the eloquent cortex with the patient sitting comfortably, which may take about an hour or even longer. After removing all metals from the patient, preparation for recording begins. First, three or more head positioning coils are placed and fixed with the tape. These coils allow us to know the exact position of the sensors in relation to the patient's head. The exact location of these coils and recording sensors are marked using a special wand called a "digitizer." After this, recording of spontaneous activity or evoked responses to various stimuli is performed. MEG recording of spontaneous activity is examined by an experienced expert to detect epileptiform activities. After visual scanning of 306 sensors, epileptiform activity is identified and further mapping of the source is performed. To map the eloquent cortex, time-locked evoked responses are evaluated and the localization of averaged responses is done in the same manner as localization of spontaneous activity. Source localization, which is a mathematical process with an assumption that there is a certain source that results in the measured magnetic field, is performed for the activities detected and this is the process of inverse problem solving. This process is finding the source of activity from the recorded magnetic flux at the surface. To solve this problem we define the source as an overdetermined single dipole (such as equivalent current dipole method) or underdetermined distributed source. When the exact location of the source is mapped and superpositioned in the patient's own brain magnetic resonance imaging (MRI), it is called magnetic source imaging (MSI)4) (Fig. 2).
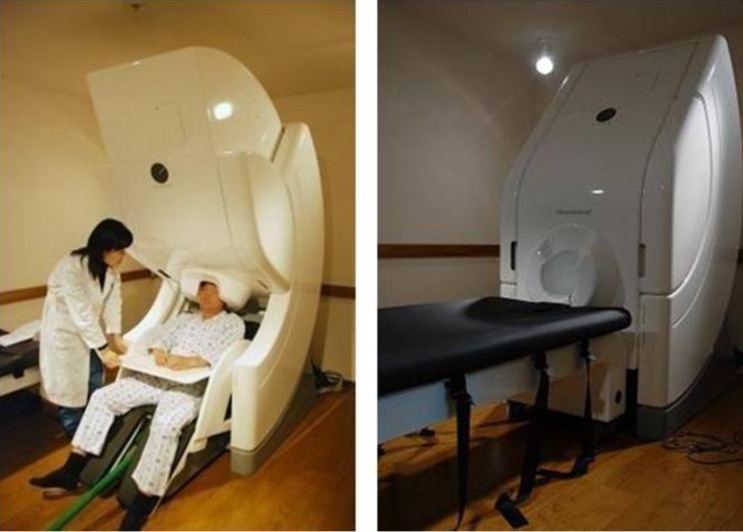
Photographs of Vectorview (Elekta Neuromag Oy), helmet-shaped, 306-channel, whole-head neuromagnetometer installed in a magnetically shielded room at Seoul National University magnetoencephalography Center. The examination can be performed with the patient in a supine or a sitting position.
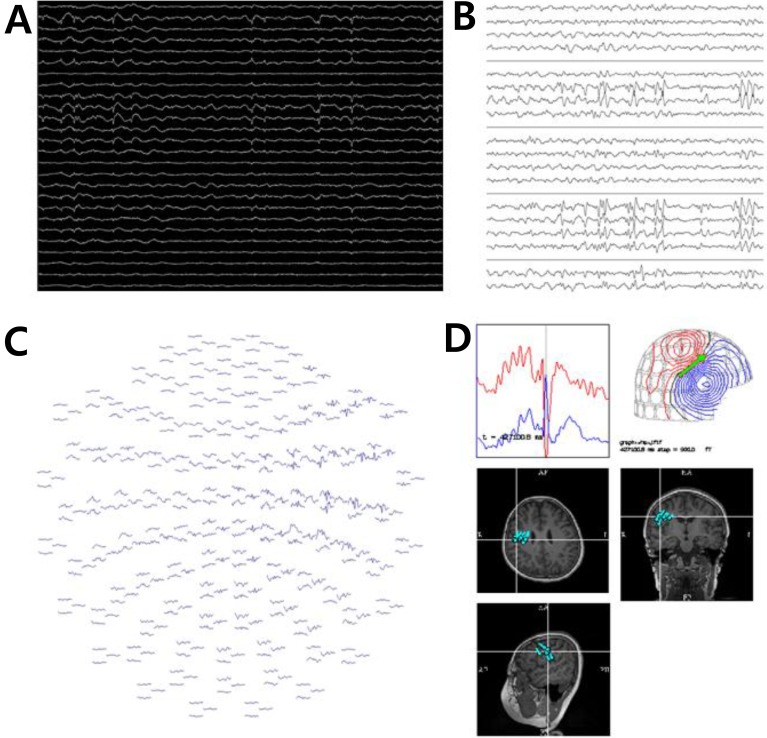
(A, B) Magnetoencephalography (MEG) data interpretation. Both MEG and electroencephalography (EEG) recordings are reviewed. Right parietal and central area MEG signals (A) and EEG recordings shown in longitudinal bipolar montages (B) from atypical benign childhood epilepsy with centrotemporal spikes are reviewed. (C) Centrotemporal spike is seen in the whole head 306 channel view. (D) Spike source localization if performed using Neuromag software and superpositioned in the patient's magnetic resonance imaging (magnetic source imaging).
4. Comparison of EEG and MEG
One of the most important strengths of MEG is that the magnetic fields are not distorted or attenuated by the different conductivity of materials that lie between the brain and the recording device (such as meninges, cerebrospinal fluid, skull, and scalp)5,6). While EEG detects secondary volume currents of extracellular postsynaptic ionic potentials, MEG detects the magnetic field flux directly generated by postsynaptic intracellular currents in the dendrites of neurons7,8). EEG is a representation of the potential difference on the scalp generated by volume currents that is a temporal and spatial summation of excitatory and inhibitory postsynaptic potentials. MEG is a direct measure of the sum of direct intracellular currents. The presence of hundreds of sensors and accurate information regarding the location of sensors allow us to localize the source with precision. In short, MEG is a direct measurement of neuronal activity with excellent temporal and decent spatial resolution.
Although the magnetic field flux generated by an electrical current is detected by MEG, the specific electrical activity produced by neurons with radial orientation to the surface is not detected by the sensors which cover the surface around the brain. EEG has its limitations in that the signal is distorted and it is much more difficult to accurately identify the source of the activity9,10). This is the reason why both MEG and EEG should be recorded simultaneously and interpreted together. In short, MEG detects the electrical activity of tangentially oriented dipoles sensitively and clearly. Localization of the source is more accurate with MEG than EEG. However, EEG detects activities generated by both radial and tangential dipoles which provide more complete information of cerebral electrical activity but less precise localization. MEG and EEG provide complementary data and it should not be considered as mutually exclusive11) (Table 1).
Application of MEG in pediatric epilepsy
There are 2 major areas of clinical application of MEG in pediatric epilepsy, which is evaluating abnormal and normal activities of the brain. MEG is used for detecting epileptiform activities with further localization of these activities and identifying the location of the eloquent cortex, which has brain functions such as motor, sensory, and language. Identifying the exact epileptogenic zone is a key to successful epilepsy surgery, but it is a theoretical zone which cannot be identified with current technology. So, currently available modalities are used and the irritative zone using EEG or MEG is defined. Another important issue in epilepsy surgery is the preservation of important functions and this is achieved by the exact localization of the eloquent cortex.
1. Localization of spike sources
Sensitivity of MEG in localization of clinically significant epileptiform activity was 79.8% (among 113 patients) which was similar to that of video-EEG (71%)12). From the largest case series of 455 patients, the average sensitivity of MEG was 70%13). MEG detected more spikes than EEG in neocortical epilepsy14), comparable spikes in anterotemporal epilepsies15), and less spikes in mesiotemporal epilepsies16). From a study that reviewed 25 studies of MEG localization, the success rate of MEG in detecting epileptiform activity was averaged to about 75%15). From the studies that estimated the accuracy of EEG and MEG for dipole source localization, there was an accuracy of 2 to 4 mm for MEG17,18). When the accuracy was compared with data from intracranial EEG, it was similar to electrocortigography19-21) and this was also the case for pediatric patients22). Knowlton et al.23) compared the localization accuracy of other modalities such as MSI, ictal single photon emission computed tomography (SPECT), and positron emission tomography (PET) and showed that sensitivity and specificity were better in MSI than ictal SPECT or PET. Although most MEG studies show interictal epileptiform activity and represent the irritative zone, a recent study by Fujiwara et al.24) identified that the interictal MEG activities showed 63% concordance with ictal MEG onset.
The advantage of MEG is considered to result from better signal to noise ratio and capability of accurate localization (few hundred sensors) and the limitation from a deeper source and radial orientation of the source25,26). To date, it is considered that MEG detection of epileptiform activity is fairly satisfactory and it has certain advantages over EEG. However it is crucial to remember that in certain cases such as mesial temporal lobe epilepsy and sources that are located deeper, MEG has a significant weakness over EEG. Iwasaki et al.10) showed very important results by blinded simultaneous interpretation of EEG and MEG. In their study that reviewed MEG and EEG from 43 patients with intractable focal epilepsy, spike localization showed similar consistency with epilepsy diagnosis in 78.1% (MEG) and 85.2% (EEG) of those patients. They concluded that MEG showed comparable but not superior to EEG in identifying epileptiform activities so that both MEG and EEG should be recorded simultaneously.
2. MEG for lesional epilepsy surgery
Localization of the irritative zone in lesional epilepsy has been shown in different pathologic substrates of epilepsy such as focal cortical dysplasia22,27-29), tuberous sclerosis30,31), and polymicrogyria32). An earlier study revealed the intrinsic epileptogenecity of focal cortical dysplasia with MEG and EEG27). Complete removal of MEG spike source clusters at the lesion and the extending clusters resulted in seizure control and good surgical outcomes22,27). MEG spike source clusters were closely related to the epileptogenic zone33) and this should be confirmed with seizure control after a successful surgery. These findings were also observed in Korean patients with lesional epilepsy surgery34). Lobar concordance was 100% with MEG spike source clusters and these clusters were located at the lesion or in the vicinity in most of the cases (Fig. 3A, B). MEG was also useful in identifying the epileptogenic tubers in patients with tuberous sclerosis35). From these results, we can define the role of MEG in lesional pediatric epilepsy surgery as identifying the epileptogenic lesion directly or indirectly and providing helpful information in planning the surgery.
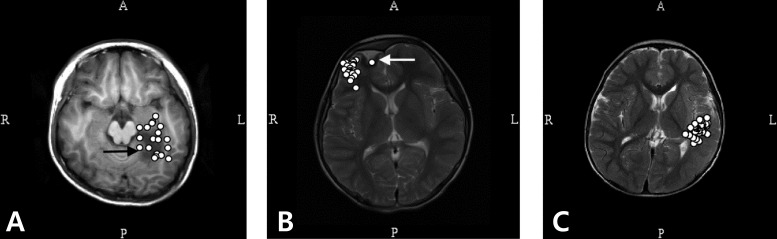
Magnetic source imaging of patients with lesional (A, B) and nonlesional (C) intractable focal epilepsy. (A) Magnetoencephalography (MEG) spike sources cluster around the lesion (focal cortical dysplasia). (B) MEG spike sources are clustered eccentric to the lesion (low grade ganglioglioma). (C) MEG spikes sources are clustered in the middle and inferior temporal gyrus in a 5 year-old patient with intractable focal epilepsy. The spike sources are overlaid in a single axial image in all imagings. R, right; L, left; A, anterior; P, posterior.
3. MEG for nonlesional intractable epilepsy
It is well known that about 20% to 40% of patients with epilepsy do not successfully respond to anticonvulsant treatment and result in pharmaco-resistant epilepsy36). In patients with pharmaco-resistant focal epilepsy, epilepsy surgery is considered as a feasible therapeutic option. To find a candidate who can benefit from epilepsy surgery, various presurgical examinations are performed including interictal EEG, long-term video-EEG monitoring, ictal SPECT, and interictal fluorodeoxyglucose-PET. MEG added information regarding epileptogenic focus in 40% of the 82 patients in presurgical evaluation. When compared to a video-EEG, MEG showed similar localization value and it was superior to interictal scalp EEG (72% vs. 60%)37). Minassian et al.38) compared MEG localization to intracranial EEG and showed that in 10 of the 11 patients, MEG spike source corresponded to the ictal onset zone identified by intracranial EEG recording. For presurgical evaluation in patients with intractable nonlesional epilepsy, MEG can provide critical information regarding the irritative zone and recruit more patients to surgery, especially whose evaluations which failed to prove localization or even lateralization to either hemisphere.
There are only a few studies that give an answer to the question whether MEG data improves surgical outcomes. The impact of MEG in 62 patients who underwent surgery, the positive predictive value for seizure freedom was 78% and the negative predictive value was 68%39). MEG showed a better predictive value than interictal PET in extratemporal neocortical epilepsy but the overall diagnostic value was higher with ictal SPECT. Ramachandrannair et al.40) evaluated MEG data and postsurgical outcomes from 22 pediatric patients whose MRI showed no lesions or interictal scalp EEG showed subtle or nonfocal findings. They reported good surgical outcomes with the inclusion of MEG spike source clusters within the resection area. However, there is insufficient evidence that interictal MEG can replace intracranial EEG monitoring41). A recent study provided promising results that MEG ictal onset localization showed fair concordance with the intracranial ictal onset zone, and the authors concluded that source localization for ictal MEG onset proved to be a useful tool for presurgical evaluation in a pediatric population with medically intractable epilepsy24).
The role of MEG in nonlesional epilepsy surgery is to assess operability in intractable epilepsy patients whose previous studies failed to show focal features. There is evidence that MEG has led to further reevaluation of high-resolution MRI42) and we frequently experience finding undetected lesions after adding long-term video EEG and MEG data in the interpretation of the MRI findings. In practice we encounter patients with intractable nonlesional epilepsy with localized MEG spike source clusters. An illustrative case of a 5 year-old boy who underwent a successful cortisectomy and is now seizure-free for 3 years is shown in Fig. 3C. MEG source localization aids in electrode positioning for intracranial EEG or electrocorticography43,44), and this may lead to sufficient coverage with grid electrodes and thus accurate identification of the epileptogenic zone.
4. MEG for mapping the eloquent cortex
As well as efficient seizure control, preservation of important functions of the brain is another important issue in epilepsy surgery. Eloquent cortices such as language, motor, and sensory should be protected to prevent paresis, aphasia or visual loss and this becomes a critical issue if the epileptogenic zone is found close to these areas. Although the gold standard method for functional mapping remains to be intraoperative cortical simulation during waking, it is impossible to perform an awake surgery in pediatric patients. An evoked response measured with MEG has excellent temporal resolution and also decent spatial resolution which is due to more than hundreds of sensors covering the whole head. Especially if this information is mapped in the patient's own brain MRI, which is an MSI, it provides a precise location of the certain evoked activity in a spatial resolution of millimeters. When compared with intraoperative stimulation, 90% concordance was shown with MEG in detecting somatosensory areas45,46). Successful mapping of the primary motor cortex was performed in pediatric patients47,48). These data provide useful information and help guide localization in epilepsy surgery49,50). There was a recent case series that performed successful passive language mapping and epilepsy surgery without complications51). Somatosensory-evoked fields were recorded successfully in 9 patients with epilepsy who were younger than 4 years with total intravenous anesthesia52). MEG is a totally noninvasive method with excellent temporal and spatial resolution in mapping eloquent cortices. These examinations can be performed safely and effectively in younger children. Results from MEG mapping of the eloquent cortex can be used as a guide when planning epilepsy surgery.
5. MEG for pediatric epilepsy other than epilepsy surgery
Although MEG is used more frequently in patients undergoing epilepsy surgery, a number of studies is increasing in epilepsy patients not undergoing surgery. These studies are more focused on identifying the difference in function and basic patho-mechanism underlying the disease. When patients with good and poor outcome groups were compared, there was a significant difference in MEG spike source, in patients with sensory motor seizure53) or peri-rolandic spike54). MEG findings were different in patients with typical benign childhood epilepsy with centrotemporal spike (BCECTS, also known as benign Rolandic epilepsy) group and those with atypical BCECTS55). Using the excellent spatial resolution of MEG, it was found that some patients with generalized 3-Hz spike-and-wave complexes had the possibility of focal onset initiating the seizure56). Since MEG is a completely contactless method, and it is readily used in detecting high frequency oscillations, whereas scalp EEG has limitations due to muscle artifact. Ictal MEG high frequency oscillation was successfully identified with MEG57), and focal onset of epileptic spasms was seen in certain patients with MEG58). More and more studies regarding patho-mechanisms of epilepsy and related cortical functions are studied and revealed with MEG, and this suggests a promising future of basic and clinical epilepsy research.
Limitations of MEG
It is crucial to notice the limitations and weaknesses of MEG to accurately interpret the data and correctly apply this information in clinical decision making. Detection of epileptiform activity with purely radial orientation was impossible with MEG and the information about epileptiform activity gathered by MEG is less complete than that of EEG. So, it is a common practice to record and interpret MEG with EEG simultaneously. There are a few studies that tried to define the MEG spike59), so there are not strict criteria for the definition. Therefore, more studies are needed to define MEG epileptiform activity for standardized interpretation and research. Although there is a report of recording ictal activity with MEG24), spike source localization provides only the information regarding the interictal activity (irritative zone vs. ictal onset zone). It is crucial to understand that the source localization is based on a certain hypothesis for a specific mathematical algorithm which is involved in solving the problem (finding the source of activity). The accuracy of this localization is expressed as probability, and the most important hypothesis is that there should be no errors with the algorithm used for the solution. Due to the unique and complex technical property and methodological aspects, it is very important that MEG should be performed and interpreted by an expert who is familiar with MEG and who has sufficient experience with MEG data interpretation. When interpreting the data, one should always keep in mind the strengths and weaknesses of MEG and the process involved in source localization. MEG recording is sensitive to movements because the sensors are fixed in the helmet and the patients head is freely movable inside the helmet. Therefore it is not easy to perform MEG in patients who cannot cooperate or we need certain amount of sedation in such patients. In the same manner, it is difficult to perform lengthy recordings event in adult patients which makes MEG less suitable for identifying ictal findings.
Conclusions
MEG is a useful tool in the evaluation of epilepsy. The most remarkable strengths of MEG over other evaluation modalities are excellent temporal and spatial resolutions. It helps to identify the irritative zone with great precision in both lesional and nonlesional epilepsies. MEG can increase the number of patients who can proceed to surgical treatment. It also improves targeting the epileptogenic zone in certain patient populations. MEG functional mapping is promising in pediatric patients who are not capable of intraoperative cortical mapping. It is more widely used in both basic and clinical research of epilepsy. Understanding the technical aspects and the limitations of MEG is critical in the application of MEG which is a technically advanced modality.
Acknowledgments
The authors thank Mrs. Mi Sun Park for her English correction of the manuscript. We are also grateful to Ms. Woorim Jeong for her help in preparation of MSI images.
Notes
No potential conflict of interest relevant to this article was reported.