A review of vitamin D deficiency and vitamin D receptor polymorphisms in endocrine-related disorders
Article information
Abstract
The endocrine system is a complex network of glands that produce and release hormones that regulate various physiological processes. In the past few decades, the human skin has been identified as an important peripheral endocrine organ that is the main site for the synthesis of vitamin D through exposure to sunlight. Mutations in downstream vitamin D-related gene pathways are associated with disease development. The vitamin D receptor (VDR) gene, which regulates the pleiotropic effects of vitamin D, has been extensively studied in adult populations. Several studies have reported the prevalence of vitamin D deficiency in children and adolescents. With changes in socioeconomic status and lifestyle, vitamin D-deficient individuals are prone to developing the disease at a young age. However, geographical and racial differences affect the association between VDR gene polymorphisms and vitamin D endocrine disorders, explaining the nonconsensus effects of polymorphisms and their association with disease development across populations. In this review, we discuss the connection between the vitamin D endocrine system and polymorphisms in the gene encoding VDR in children and adolescents, focusing on its effects on growth, puberty, insulin resistance, and the immune system.
Key message
· The prevalence of vitamin D deficiency is high among child ren and adolescents and mainly attributed to changes in environmental factors.
· Vitamin D hormonelike properties are associated with many endocrinerelated disorders.
· The effect of vitamin D is modulated by the vitamin D re ceptor, polymorphisms of which are reportedly as sociated with an increased risk of disease development in children and adolescents.
Introduction
The endocrine system consists of numerous glands throughout the body that produce and secrete hormones that regulate several physiological processes [1]. Hormones are transported via the blood to their target cells. Some hormones affect only a few specific target cells, whereas others affect numerous cell types throughout the body. The target cells for each hormone are characterized by the presence of specific hormone receptors located on or inside the cell. The interaction between the hormone and its receptor triggers a cascade of biochemical reactions in the target cells, eventually modifying their function or activity [2]. In the past few decades, the human skin and its appendages have been identified as endocrine organs and are the major target tissues for hormones [3]. The skin is able to generate hormones and substances with hormonelike activities. These substances appear to exert pleiotropic effects via paracrine, autocrine, intracrine, and endocrine mechanisms [4].
The skin is a unique site where vitamin D3 is produced. It is then converted by the liver to 25-hydroxyvitamin D3 (25(OH)D), which is further converted to 1,25-dihydroxyvitamin D3 (1,25(OH)2D) (calcitriol) in keratinocytes [3]. Vitamin D insufficiency affects approximately 50% of the global population. An estimated 1 billion people worldwide across all ethnicities and age groups have a vitamin D deficiency (VDD) [5]. This VDD pandemic been attributed to sociodemographic [6], lifestyle, and environmental factors [5,7]. Certain populations are at higher risk of developing VDD than the general population, namely infants, children, pregnant women, and women of childbearing age, owing to their increased metabolism and dietary need for vitamin D. Interestingly, some case series have reported a possible association between VDD and the coronavirus disease 2019 pandemic considering stay-at-home orders and inadequate sunlight exposure [8,9]. The prevalence of severe VDD among the young is inconsistent, but it is much higher in some countries, especially in the Middle East and Southeast Asia [7]. Several studies highlighted the strong association between VDD and chronic and acute diseases [10]. There is increasing evidence that VDD in children and adolescents contributes to the development of non-communicable diseases in adulthood [11]. Moreover, the large amount of observational data currently available is accompanied by pathophysiological associations between vitamin D and energy homeostasis and regulation of the immune and endocrine systems [10].
Calcitrol (1,25(OH)2D) serves as a high-affinity ligand for VDR in the target tissues and modulates the expression of vitamin D–directed genes [12]. Its local production is responsible for the regulation of up to 200 genes that may facilitate pleiotropic health benefits [5]. VDR is found in endocrine glands and cardiovascular tissues, such as endothelial cells, vascular smooth muscle cells, and cardiomyocytes. VDR is also found in hematopoietic cells, and vitamin D has been shown to regulate cell differentiation and interleukin and cytokine production. Considering the near-universal expression of VDR across tissues, vitamin D is expected to play a role in diseases other than bone diseases. The wide variety of non-skeletal diseases in which vitamin D is important include cardiovascular diseases, obesity, metabolic syndrome (MetS), insulin resistance (IR), infection, allergies, some forms of cancer, and autoimmune diseases [13]. Ongoing research is exploring the potential association between vitamin D levels and certain diseases including cancer [14]. Many of these effects are associated with vitamin D as an endocrine, autocrine, and paracrine biochemical signal that acts on almost any tissue or cell type. Children and adolescents are particularly vulnerable to VDD owing to bone growth and bone mass acquisition, which are characteristic of these phases. In addition, new data have shown the negative consequences of deficient or insufficient serum vitamin D levels in relation to other morbid conditions beyond bone and mineral metabolism [14].
Methodology
Here we discuss the association between the vitamin D endocrine system and polymorphisms in the genes encoding vitamin D receptors in children and adole scents. A literature search was conducted of the PubMed, Scopus, Science Direct, and Google Scholar electronic databases over a 20year period (2003–2023). The inclu sion criteria were as follows: (1) vitamin D endocrine system; (2) vitamin D receptor gene polymorphisms; (3) children/adolescents; (4) growth and puberty; (5) IR; and (6) autoimmunity. The search terms included "vitamin D" AND "endocrine system," "vitamin D/vitamin D receptor gene" AND "child ren and adolescents," "vitamin D/vitamin D receptor gene" AND "growth/puberty," "vitamin D/vitamin D receptor gene" AND "insulin resistance," and "vitamin D/vitamin D receptor gene" AND "autoimmunity." The search was limited to publications in English. The titles and abstracts of the selected studies were screened, and then the full texts of potentially relevant articles were retrieved.
Vitamin D
Vitamin D is a fat-soluble steroid-derived vitamin distributed throughout various tissues of the body [15]. There are 2 natural forms of vitamin D: ergocalciferol (vitamin D2), which is synthesized by plants, and cholecalciferol (vitamin D3), which is synthesized by mammalian skin [14]. Vitamin D is mainly the result of endogenous synthesis and often considered more of a hormone than a vitamin [16]. During sunlight exposure, ultraviolet B (UV-B) radiation at wavelengths of 290–315 nm17) is absorbed by the immediate precursor of cholesterol, 7-dehydrocholesterol, in the skin [18]. UV-B-mediated photolytic and nonenzymatic reactions convert 7-dehydrocholesterol to previtamin D3. Previtamin D3 undergoes nonenzymatic thermal isomerization to vitamin D3 [12]. Once formed, previtamin D3 and vitamin D3 continue to absorb UV radiation over a wide range of wavelengths, resulting in their breakdown into biologically inert photoproducts. Therefore, a steady state is reached after prolonged exposure to UV radiation, in which only 10%–15% of cutaneous 7-dehydrocholesterol is converted to previtamin D3. This photoregulatory process is thought to ensure that toxic levels of vitamin D3 are not synthesized under excessive sun exposure conditions [19]. Once formed, vitamin D3 is believed to diffuse from the epidermis into the dermal capillary bed, where the vitamin D-binding protein rapidly binds it for transport to the liver [18], where it is converted by one of several high-capacity cytochrome P450s to 25(OH)D, a prohormone or immediate precursor metabolite of the active form of vitamin D, 1,25(OH)2D [12]. The product of a single enzyme, mitochondrial 1-α-hydroxylase (CYP27B1), 1,25(OH)2D serves as a high-affinity ligand for the VDR in target tissues, where it modulates the expression of vitamin D–directed genes [12]. The biological effects of 1,25(OH)2D are initiated by binding of the hormone to a cytosolic receptor in the target organs. The 1,25(OH)2D receptor complex is tightly associated with the cell nucleus and stimulates deoxyribonucleic acid (DNA) transcription to synthesize messenger ribonucleic acid (mRNA) and translate proteins that perform its biological action [20]. As a lipid-soluble molecule, vitamin D is taken up by adipocytes and stored in the subcutaneous or omental fat deposits for subsequent use. The distribution of vitamin D in the adipose tissue prolongs its total body half-life by approximately 2 months [19]. In addition to its role in bone metabolism, vitamin D has multiple beneficial extraskeletal effects. Vitamin D plays a role in glucose homeostasis, insulin secretion and action, lipolysis regulation [21], endocrine disease pathogenesis, immune competence modification, blood pressure regulation, cancer and infectious disease risk modulation, and autoimmune disease propensity [22].
Prevalence and associated factors of VDD in children and adolescents
VDD, a major public health problem and an unrecognizable epidemic in developed and developing countries, has a prevalence of 30–93% in children and adults [21]. A previous study suggested that the “normal” concentration of circulating serum 25(OH)D was at least 32 ng/mL [23]. The Institute of Medicine/National Academy of Medicine proposed that a level of 16 ng/mL (40 nmol/L) meets the needs of approximately half of the population (median population requirement or estimated average requirement), and at least 20 ng/mL (50 nmol/L) meets the needs of at least 97.5% of the population (akin to the recommended dietary allowance) [24]. Table 1 shows the vitamin D cutoff values according to 25(OH)D concentration [25-27]. The differences in cutoff values suggest that they vary between countries and vitamin D target organs. According to the Vitamin D Supplemental Guidelines by Pludowski, a vitamin D level of <20 ng/mL (<50 nmol/L) in adolescents has clinical implications that increase the risk of bone health-related problems and cardiovascular diseases in adulthood [28].
Epidemiological data are limited on vitamin D inadequacy in children in most countries. However, available data have shown that VDD affects 10%–80% of Asian infants, children, and adolescents living in tropical and subtropical countries [24]. A recent study applying the Vitamin D Standardized Program protocol in 14 European populations reported that 13% of 55,844 individuals presented with mean serum 25(OH)D concentrations <30 nmol/L regardless of age group, ethnicity, or latitude [29]. Al-Othman et al. [30] revealed that 11.4% of children and adolescents in Saudi Arabia had severe VDD, whereas 71.6% and 16.8% had moderate and mild deficiencies, respectively. These findings are interesting considering that these countries receive year-round sunlight exposure. Several studies have reported a higher prevalence of VDD in female subjects [22,24,31-34]. Environmental factors, such as air pollution, may play a contributory role. Tropospheric ozone, an efficient absorber of UV radiation, is a common air pollutant found in urban areas and may indirectly reduce the availability of sunlight to urban populations [33]. The scattering and absorption of light by clouds may not significantly reduce natural light exposure because over 90% of UV rays can penetrate clouds [25]. However, season seems to be more important than latitude, although both account for less than one-fifth of the observed variation in serum 25(OH)D levels [14].
It was observed that the serum concentration of 25(OH) D, a marker of vitamin D nutritional status, was highest in autumn, followed by spring and winter in a multicenter cross-sectional study of 1,006 European adolescents [35]. There is a well-documented seasonal cycle of vitamin D status in latitudes with clear seasonal changes in solar UV availability, with a maximum in late summer and a minimum in late winter [36,37]. As the zenith angle of the sun becomes more oblique, the length of the path traversed by UV-B photons increases. Consequently, ozone, which efficiently absorbs UV-B radiation, absorbs all UV-B photons before they reach the Earth’s surface. This explains why previtamin D3 production varies at different latitudes, times of day, and seasons [18]. However, the assumption that vitamin D levels depend on latitude gradients might not be true, as geophysical surveys have shown that UV-B penetration over 24 hours during summer in northern Canadian latitudes is equal to or exceeds that at the equator [25].
Approximately 23% of the variability in serum 25(OH) D concentrations is associated with race or ethnicity [38]. The skin color of indigenous races darkens as latitude decreases from the poles to the equator and ambient UV light increases [37]. In Italy, non-white adolescents had an 8-fold higher risk of VDD and a 6-fold higher risk of severe deficiency than white adolescents [36]. Similarly, in Malaysia, VDD in adolescents is higher among Indian and Malay ethnicities than among Chinese individuals, suggesting an inverse correlation between the production of vitamin D precursors following exposure to UV radiation and skin melanin density [28,30,39]. Tsiaras et al. [19] reported that individuals with darker skin pigmentation or higher melanin content require longer sun exposure than those with lighter skin to produce the same amount of vitamin D required by the body. This may be partially due to differences in melanin content, as melanin competes with UV-B radiation, resulting in a higher threshold for UV-B-induced cutaneous vitamin D production in darker skin [40]. However, there are few studies on dark-skinned subjects (Fitzpatrick phototype IV), who tend to be mostly vitamin D sufficient [41]. Bogh et al. [42] measured baseline serum 25(OH)D and total cholesterol (TC) levels in 182 fair- and dark-skinned subjects and studied the effect of UV radiation on serum 25(OH)D levels. They found that the amount of serum 25(OH)D produced was determined by the amount of cholesterol in the skin rather than skin pigmentation [42]. Matsuoka et al. investigated the effect of racial pigmentation on vitamin D3 formation by simulating the process with a fixed dose of UV-B radiation and concluded that, although racial pigmentation has a photoprotective effect, it does not prevent the generation of normal levels of active vitamin D metabolites. Individuals with dark skin compensate for low 25(OH)D levels by rapidly converting it to the active 1,25(OH)2D metabolite, allowing them to maintain an adequate vitamin D status [25].
A high prevalence of VDD has been reported among children in tropical countries regardless of the availability of sunlight. Sun-seeking behavior is uncommon in these populations because of their hot climates. Thus, sun protective behaviors, including wearing a hat, applying sunscreen, using an umbrella, wearing long sleeves, and staying in the shade influence vitamin D status [43]. Although Malaysia is a tropical country with ample sunshine throughout the year, the prevalence of VDD (<50 nmol/L) in children is high at 47%–75% [28]. Children do not seem to receive enough sun exposure as they tend to spend more time indoors than outdoors [32]. In certain countries, women receive little or no sunlight because they cover their bodies with dark veils for cultural and religious reasons [30,32,34,39]. Apart from sunscreen, clothing is an obvious physical barrier between solar radiation and the skin, which has been specially designed to prevent UVB radiation (and, more recently, UV-B and UV-A radiation) from reaching the skin. It is well-established that sunscreen markedly reduces the transmission of UV-B radiation to the skin while proper application of sun protection factor (SPF) 15 sunscreen reduces vitamin D synthesis by 99.9% versus 97.5% for SPF 8 [37]. Contradictory information was provided by Diehl and Chiu, who concluded that, although sunscreens are effective, many may not block UV-B due to improper application. Therefore, sunscreen use may not diminish vitamin D synthesis in a real-world setting. However, prolonged exposure to unprotected sunlight should be avoided to reduce the risk of developing skin cancer [25].
Vitamin D receptor
In addition to environmental contributions, genetic factors may account for 23%–80% of the variability in serum vitamin D levels, as observed in twin studies [44]. The genomic actions of 1,25(OH)2D are mediated by VDR, which belongs to the family of steroid hormone zinc fingers [45], a transcription factor [46] located on chromosome 12 (12q13.11), and consists of 11 exons spanning 63,495 bp [47]. In addition to 1,25(OH)2D, VDR regulates the transcription of target genes via heterodimerization with the retinoid X receptor (RXR). The VDR-RXR complex translocates to the nucleus and interacts with DNA sequence elements or vitamin D response elements in the promoter regions of target genes [45,46,48]. The vitamin D system has pleiotropic functions and regulates approximately 3% of the human genome [49], calcium/phosphate homeostasis, cellular proliferation and differentiation, and the immune response in a ligand-dependent manner. VDR is present in the peripheral adipose tissue, small intestine, bone, and kidneys, all of which play major roles in regulating calcium and phosphorus metabolism. The skin, brain, gonads, colon, β-islet cells, prostate, heart, skeletal muscle, monocytes, activated T and B lymphocytes, and adipocytes express the same VDR [31,45]. Genetic alterations in the VDR can lead to defects in gene activation, cell proliferation and differentiation, calcium homeostasis, and other related biological mechanisms [48]. Different VDR gene polymorphisms may interfere with the concentration and, especially, the action of vitamin D. To date, BsmI, FokI, ApaI, and TaqI are VDR gene polymorphisms that have been extensively investigated under various clinical conditions [50]. FokI, which is located in the coding exons, regulates VDR protein length and activity as a transcription factor. In contrast, BsmI, ApaI, and TaqI polymorphisms in the 3'-untranslated region are associated with the regulation of mRNA stability and half-life, resulting in a better response to vitamin D in the target tissue [51]. VDR expression and its transactivation of human cathelicidin-like antimicrobial protein are influenced by the FokI genotype and, together with ethnicity, influence 1,25(OH)2D-elicited CYP24A1 expression and vitamin D levels [28]. The frequency of these polymorphisms depends on ethnicity and varies between Asians and Caucasians as well as among Asian countries [52].
In Brazil, the wild-type BsmI, ApaI, and TaqI genotypes were significantly associated with lower 25(OH)D levels. Compared with the wild-type genotype, 25(OH)D deficiency was less frequent in the BsmI GA+AA and TaqI TC+CC genotypes [47]. However, Cobayashi et al. [38] reported that the BsmI mutant T allele is associated with an increased risk of low serum 25(OH)D concentrations. The Ht1 haplotype of BsmI, ApaI, and TaqI (GGT) was considered the risk haplotype, whereas the Ht7 haplotype (ATC) was considered the protective haplotype against circulating 25(OH)D levels [47]. Moreover, the Ht1 haplotype of VDR was similar to that found in Caucasians and Asians, followed by Ht7 and Ht3 (GTT) as found in Caucasians, showing strong linkage disequilibrium among these 3 single nucleotide polymorphisms (SNPs) [47]. In Kazakhstan, Zhumalina et al. [53] observed a 1.83-fold reduction in serum 25(OH)D levels in children with the FokI C allele, whereas children with the CC genotype showed a 2.3-fold reduced serum 25(OH)D level. It is likely that the FokI CC genotype alters its role in the metabolic feedback loops or affects the rate at which 25(OH)D is metabolized. It can be speculated that the FokI genotypic variants require a higher threshold 25(OH)D level to exhibit feedback effects. Alternatively, this SNP may be in linkage disequilibrium with neighboring functional polymorphisms known to affect baseline 25(OH)D levels [54]. The BsmI AA genotype featured a lower vitamin D concentration than the GG and GA genotypes. However, the distribution of the BsmI and FokI polymorphisms was independent of vitamin D levels [53]. Girls with the CC/GG genotypes -1521 (G/C) and -1012 (G/A) of the human VDR promoter (hVDRp) had lower circulating levels of 25(OH)D and tended to have higher circulating 1,25(OH)2D levels. Similarly, low-normal 25(OH)D levels and elevated 1,25(OH)2D levels were observed in children with genetically altered VDR function and severe vitamin D resistance with no detectable consequences on calcium metabolism or lower serum insulin-like growth factor (IGF-1) levels and transcriptional activity in classical vitamin D target cell types. These findings are thought to result from increased 1,25(OH)2D production and decreased 1,25(OH)2D catabolism, both of which are dependent on VDR. Both SNPs were previously shown to cause dramatic changes in protein-DNA complexes [55].
Vitamin D endocrine system
The steroid hormone 1,25(OH)2D generates biological responses by regulating gene transcription and rapidly activating various signal transduction pathways at or near the plasma membrane. Genomic responses to 1,25(OH)2D result from its stereospecific interaction with its nuclear receptor, the VDR nucleus [56], within organs such as the bones, parathyroid, and immune system [51], Production of the vitamin D endocrine hormone 1,25(OH)2D is regulated by habitual dietary calcium intake and physiological states such as growth, aging, and menopause [57]. In the endocrine portion of the vitamin D system, the last step occurs in the kidney and is responsive to controls such as the parathyroid hormone (PTH) and fibroblast growth factor 23 (FGF23) [58].
PTH is the principal regulator of renal 1,25(OH)2D synthesis. The renal production of 1,25(OH)2D is tightly regulated, and serum 1,25(OH)2D levels change very little in response to vitamin D challenge [59]. The ability of the vitamin D endocrine system to produce the steroid hormone, 1,25(OH)2D depends on the circulating concentration of 25(OH)D, which is tightly regulated by CYP27B1 [56,60]. In addition, 1,25(OH)2D concentrations were deemed positively associated with serum PTH levels, consistent with the well-known effect of PTH in upregulating CYP27B1 and increasing the conversion of 25(OH)D to 1,25(OH)2D in the kidneys [60]. In contrast, a negative association was observed between serum 25(OH)D and PTH levels [36,60], with pronounced variation between summer and winter [17,36]. The cutoff point for serum 25(OH)D at which the mean serum PTH concentration began to increase was defined as 20 or 30 ng/mL (50–75 nmol/L) [61]. Moreover, interventional studies have shown that intestinal calcium absorption decreases when 25(OH)D serum levels are <4 ng/mL (10 nmol/L), while calcium absorption plateaus when serum 25(OH)D levels are ≥8 ng/mL (20 nmol/L), suggesting that calcium absorption VDD is reduced secondary to a substrate 25(OH)D deficiency and 1,25(OH)2D production [62]. PTH levels are primarily regulated by ionized calcium rather than directly by vitamin D levels [17]. Calcium and phosphorus are absorbed in the upper small bowel via an active transcellular transport mechanism stimulated by 1,25(OH)2D [60,63]. During rapid growth in infancy and early childhood, calcium and phosphorus absorption are important for bone mineralization [63]. When intestinal calcium absorption is insufficient, hormonal adaptations release calcium from the bones to ensure normocalcemia, not only by increasing bone loss but also by decreasing bone mineralization [62]. FGF23 is a hormone secreted by osteocytes in the bone, which, together with its co-factor α-Klotho, inhibits phosphate reabsorption and 1,25(OH)2D production in the kidneys [64]. Vitamin D importance in endocrine system potentially associated with development of endocrine related disease. Summaries of their association were shown in Table 2.
Vitamin D increases the expression of insulin receptors in vitro, thereby enhancing insulin responsiveness for glucose transport. Vitamin D may indirectly enhance insulin action by regulating extracellular calcium, thereby affecting calcium influx through the cell membranes and maintaining adequate intracellular cytosolic calcium pools [65]. Insulin secretion is a calcium-dependent process; therefore, alterations in calcium flux can adversely affect β-cell secretory function [66,67]. Vitamin D enhances insulin sensitivity by stimulating the expression of insulin receptors and/or activating peroxisome proliferator-activated receptor-δ (PPAR-δ) [65,67,68]. PPAR-δ has been implicated in the regulation of fatty acid metabolism in skeletal muscle and adipose tissue [67,68]. Changes in intracellular calcium levels in insulin target tissues may contribute to peripheral IR via impairing signal transduction pathways, leading to decreased glucose transporter activity [67]. PTH may mediate IR by reducing glucose uptake in the liver, muscle, and adipose cells [66]. Vitamin D can also indirectly affect IR through the renin-angiotensin-aldosterone system (RAAS). Ang II inhibits the action of insulin in vascular and skeletal muscle tissues, leading to impaired glucose uptake. Vitamin D suppresses renin formation and the local pancreatic RAAS [67]. Vitamin D and IGF-1 levels affect each other; specifically, an increase in vitamin D levels increases IGF-1 levels. IGF-1 stimulates CYP27B1 activity, which in turn regulates the renal production of vitamin D,1,25(OH)2D [69]. IGF-1 synthesis and secretion are regulated by growth hormone (GH) levels, whereas IGF-1 levels can generate a negative feedback loop that regulates pituitary GH secretion. IGF-1 also plays a key role in slowing glucose level increases, fat metabolism, and MetS [70]. In humans, resistin is secreted by adipocytes and macrophages in the adipose tissue and liver, likely stimulating the secretion of proinflammatory molecules [68,71] that have been proposed to play a major role in the development of IR in obesity [71].
Vitamin D may also indirectly influence lipid metabolism through insulin. Vitamin D is well-known to regulate lipid metabolism by inhibiting lipid synthesis and promoting adipopexis in adipocytes. The potential underlying mechanism operates through facilitating calcium homeostasis via vitamin D. Vitamin D increases intestinal calcium absorption by affecting the rate of fat absorption, which results from the ability of calcium to bind to fatty and bile acids, forming calcium–fatty acid soaps that are excreted in the feces. The inhibition of hypertriglyceridemia may be caused by the inhibition of microsomal triglyceride transfer protein activity via the suppression of hepatocellular calcium [72]. The role of vitamin D in the risk of hypertension has been demonstrated, indicating that vitamin D may be a negative endocrine regulator of the RAAS and key stabilizer of blood pressure balance. The downregulation of 1,25(OH)2D-mediated renin expression and RAAS activity occurred through its interaction with VDR [48].
Vitamin D enhances insulin secretion and promotes β-cell survival by modulating cytokine generation. The anti-apoptotic action of vitamin D is mediated by Fas- related pathway downregulation [67]. Vitamin D reduces major histocompatibility complex class I molecules and Fas expression (transmembrane cell surface receptor mediator), leading to the suppression of pancreatic β-cell apoptosis. It also increases A20 protein expression, which has an anti-apoptotic function against pancreatic β-cells, and increases insulin production [73]. Vitamin D may promote β-cell survival by inactivating nuclear factor κB (NF-κB) and the effects of cytokines [67]. In addition, 1,25(OH)2D protects β-cells from detrimental immune attacks by acting directly on β-cells and indirectly on different immune cells, including inflammatory macrophages, dendritic cells, and a variety of T cells [66]. Furthermore, vitamin D decreases human leukocyte antigen class I expression, reducing the vulnerability of islet β-cells to cytotoxic T lymphocytes [74]. Moreover, 1,25(OH)2D decreases the maturation of dendritic cells and inhibits the release of interleukin (IL)-12 (stimulating T-helper [Th]-1 cell development), IL-2, interferon-γ, and tumor necrosis factor-α (TNF-α) (stimulators of inflammation), which are involved in the destruction of β-cells and result in IR [66].
The detection of VDR in almost all cells of the immune system, particularly antigen-presenting cells and activated T cells, has led to investigation of the potential role of vitamin D as an immunomodulator [66]. In lymphoid tissues, antigen-presenting cells, macrophages, and dendritic cells have CYP27B1 activity and can convert ambient 25(OH)D into an active vitamin D metabolite. This factor implies that vitamin D insufficiency is associated with impaired macrophage activation and function; moreover, the local concentration of 1,25(OH)2D in lymphoid tissue can be too low to effectively suppress the Th-1-type immune response [20,75]. In addition, the activation of nuclear VDR modifies transcription via several intracellular pathways and influences immune cell proliferation and differentiation [66]. VDD is associated with increased inflammation since it attenuates the expression of proinflammatory cytokines such as IL-1, IL-6, and TNF-α and downregulates NF-κB activity [65]. Vitamin D compounds suppress T-cell activation by binding to VDR; thus, VDR gene polymorphisms may be associated with T-cell-mediated autoimmune diseases. This hormone inhibits T-cell activation in vitro and in vivo and inhibits the secretion of proinflammatory cytokines that are considered involved in the pathogenesis of autoimmune diseases [76,77]. Biological interactions have also been reported between vitamin D and cytotoxic T lymphocyte antigen 4, a critical suppressive protein that is constitutively expressed by regulatory T cells (Tregs) and induced in conventional T cells following activation. It also restrains the inappropriate activation of autoreactive T cells and restores T-cell homeostasis after activation [74]. Overall, 1,25(OH)2D directly modulated T-cell proliferation and cytokine production, decreased Th-1 cell development, inhibited Th-17 cell development, and increased Th-2 and Treg production [66].
TNF-α and IL-6 inhibit the production of adiponectin-stimulating puberty onset and inhibit gonadotropin-releasing hormone (GnRH) release, resulting in delayed-onset puberty. Leptin, which is secreted by adipocytes, acts as a permissive stimulus for pulsatile GnRH secretion. Kisspeptins released by hypothalamic kisspeptin neurons also stimulate GnRH neurons and appear to be gatekeepers of pubertal onset [78]. GnRH secreted by the hypothalamus stimulates the pituitary gland to release gonadotropins such as luteinizing hormone (LH) and follicle-stimulating hormone (FSH). At the onset of puberty, FSH stimulates the ovaries to produce estradiol (E2), which is responsible for breast tissue growth, one of the earliest clinical signs of puberty [79]. Gonadotropins stimulate gonadal maturation and sex steroid synthesis [79]. Moreover, vitamin D can activate tryptophan hydroxylase-2, which is involved in the tryptophan synthesis of serotonin and further affects melatonin synthesis. Melatonin binds to hypothalamic receptors and inhibits GnRH secretion, thereby inhibiting initiation of the gonadal axis [80].
IGF-1 modulates pubertal onset and progression by stimulating GnRH expression. Therefore, it is conceivable that vitamin D-mediated effects may influence IGF-1 levels and pubertal onset by affecting gonadotropin and sex hormone levels [81]. Furthermore, vitamin D is a hormone that regulates P450 aromatase, an enzyme capable of aromatizing androgens to estrogen that is found in fat tissue as well as osteoblasts. Vitamin D and its receptor may influence androgen–estrogen balance in the peripheral tissues, thereby changing the availability of steroid hormones for their receptors. The difference in steroid concentrations between males and females induce the sex specific effects of the VDR genotype on bone mass and predictive capacities of bone metabolism markers [82].
Endocrine-related disorders and VDD
The prevalence of VDD is higher among adolescents than prepubertal children, suggesting an effect of puberty on vitamin D levels. The physiological increase in adipose tissue during puberty decreases the bioavailability of 25(OH)D. Puberty is a critical phase in bone development during which there is an increased demand for vitamin D owing to increased bone accretion [83]. In a cross-sectional study of 384 children and adolescent in Iran, a low vitamin D serum level was observed in Tanner stage IV–V boys and Tanner stage II–III girls [83]. Lee et al. [84] observed low mean serum 25(OH)D levels in children with central precocious puberty (CPP), among whom advanced Tanner stages (Tanner stages III and IV) were more frequently observed. Decreased vitamin D levels increased the susceptibility of girls to CPP by 1.19-fold [84]. In China, individuals with new-onset precocious puberty (PP) have a mean 25(OH)D level of <20 ng/mL [80]. Gan et al. [85] recently reported that VDD is associated with an increased risk of idiopathic CPP. In that study, vitamin D levels were significantly associated with height, weight, breast stage, mother’s height, and LH/FSH ratio in Chinese girls with idiopathic CPP [85].
In contrast, in a cross-sectional study conducted in Spain, the vitamin D status of 6- to 8-year-old girls with CPP was similar to that of prepubertal girls [86]. PTH concentrations were higher among those with vitamin D levels <30 ng/mL, especially girls with CPP. Girls with PP may have elevated PTH concentrations because they appear to develop chronologically [86]. Moreover, serum 25(OH)D levels are inversely associated with bone age and chronological age [84]. VDD may affect the absorption of calcium ions, which regulate VDR, further activating the hypothalamic-pituitary-gonadal axis and leading to PP [80]. Under physiological conditions, PTH promotes bone mineralization by affecting osteoblasts. During the hypovitaminosis D or hypocalcemic phases, PTH concentrations increase and osteoclasts become their main target cells in the bone, activating bone resorption. Among healthy children and adolescents, PTH concentrations reportedly increase significantly in girls aged 8–10 years but are significantly increased in boys aged 10–12 years, leading to the hypothesis of a relationship between PTH concentrations and the pubertal and bone growth spurt. That is, the increase in PTH concentrations in these age groups, with clear sexual dimorphism, could be a physiological characteristic of the pubertal period and, in this case, PP [86].
In the CPP group, there was a positive correlation between PTH concentration and growth rate, bone age, and basal E2, basal FSH, basal LH, and peak LH concentrations [86]. LH levels were higher in children with CPP, whereas FSH, E2, leptin, and kisspeptin levels were significantly higher in children with CPP and PT than in their healthy counterparts. Melatonin levels were significantly higher in the PT group, whereas serotonin levels were significantly higher in the healthy group [80]. Several studies reported that PP is related to obesity and overweight [80]; however, this relationship is bidirectional; that is, excess weight can also influence puberty. The proposed mechanisms for these relationships include the adipose-tissue activation of aromatase, an enzyme that catalyzes the conversion of androgens to estrogens that is known to increase body fat, favoring weight gain [68]. The mechanism by which these factors lead to PP may be related to the influence of adipokines (particularly leptin and adiponectin) on the hypothalamic-pituitary-gonadal axis, expression of adipokines and their receptors in the gonads, and peripheral activities of adipose tissue such as aromatase activity [80]. The obesity gene in white fat stimulates leptin synthesis and release, while high leptin levels stimulate kisspeptin secretion [80].
Obesity, central obesity, hypertension, hypertriglyceridemia, low high-density lipoprotein cholesterol (HDL-C) level, IR, and MetS are all associated with an increased risk of a low 25(OH)D level [87]. Children with 25(OH)D deficiency were characterized by a higher occurrence of impaired fasting glucose (IFG) [15,21,65,88-91], larger waist circumference (WC) [92-94], higher triglyceride (TG) level [15,68,89,92,95,96], higher insulin level [15,68,71,93,97,98], IR [15,22,68,71,87,88,93,97-100], and higher leptin concentrations, all of which were inversely associated with 25(OH)D levels and insulin sensitivity [68,71]. Vitamin D-deficient children are twice as likely to develop prediabetes, as reflected by IFG, as those with 25(OH)D levels >20 ng/mL (50 nmol/L) [91]. In the China Child and Adolescent Cardiovascular Health Study, an L-shaped correlation between age and 25(OH)D level was observed in all children with a threshold age of 14 years. Furthermore, an inverted U-shaped association was noted between body mass index (BMI) and 25(OH)D in which fat mass index and fat mass percentage (FMP) were inversely associated with 25(OH)D concentration, particularly in boys [101]. This reduction in serum calcidiol levels, coinciding with increased adiposity, is assumed due to the enhanced sequestration of vitamin D in the fat, a factor leading to decreased bioavailability [102]. Moreover, low glucose tolerance associated with obesity is also observed in pubertal versus prepubertal children, which, in addition to the increased production of adipokines in obesity, increases their risk of developing IR during puberty [68].
In a cross-sectional study of children and adolescents aged 3–15 years, a negative correlation was observed between vitamin D and PTH levels, with a high prevalence of hypovitaminosis D and high mean PTH levels in the severe obesity and obesity groups. In fact, the mean PTH levels substantially increased as BMI increased, showing a positive association [102]. Additionally, a wealth of evidence indicates a negative correlation between BMI and vitamin D level [22,31,83,87-89,92,102-109]. The mean vitamin D level decreased substantially as BMI increased from 28.2 ng/mL in a normal nutritional situation to 23.1 ng/mL in patients with severe obesity. Similarly, mean PTH values substantively increased as BMI increased from a mean 31.1 pg/mL in individuals with a normal BMI to 45.2 pg/mL in individuals with severe obesity [102]. In a cross-sectional study of 202 children and adolescents aged 5–15 years, skinfold thickness, Homeostatic Model Assessment for Insulin Resistance (HOMA-IR) score, and fasting and post glucose insulin, PTH, low-density lipoprotein cholesterol (LDL-C), serum cholesterol, and high-sensitivity C-reactive protein (hs-CRP) levels were significantly negatively correlated with vitamin D levels [88]. Every 10 ng/mL increase in 25(OH)D level caused a mild decrease in fasting blood glucose level and HOMA-IR score [61]. Although a high prevalence of VDD and insufficiency was observed, high PTH levels were noted in only 11 (7.2%) patients, all of whom had vitamin D levels within the deficient range. This finding is consistent with the possible explanation that PTH axis activation in obesity occurs at much lower vitamin D levels than normal [88].
In a cross-sectional study in Spain, overweight or obese children with the highest levels of sex hormones, FSH, LH, and E2 also presented with low 25(OH)D levels [110]. In Ukraine, the high prevalence of 25(OH)D deficiency in adolescent females with polycystic ovary syndrome (PCOS) was related to obesity [111] through androgen-mediated premenarcheal LH excess [112]. In women with PCOS, the function of the GnRH pulse generator is perturbed, resulting in LH overproduction and a relative FSH shortage. LH oversecretion further increases androgen production by the theca cells surrounding the follicle [113]. Anti-Müllerian hormone (AMH) levels were significantly higher in adolescent females with PCOS, a finding that is consistent with previously published reports suggesting AMH “cutoffs” around 6.26–7 ng/mL [114]. The presence of 25(OH)D diminishes AMH II and FSH receptor mRNA expressions with increased progesterone secretion by primary human granulosa cells, resulting in luteinization accompanied by increased progesterone secretion that can lead to follicular atresia and apoptosis in patients with PCOS [115]. A lack of negative feedback from progesterone resulting from hypothalamic prenatal androgenization [113] causes granulosa-cell apoptosis by downregulating the aromatase enzyme, which is essential for estrogen production, by the vesicles. Moreover, VDD enables acquisition of the endometrial thickness required for embryonic implantation [115].
A cross-sectional study in Sri Lanka of 202 children and adolescents reported that hs-CRP and vitamin D levels were significantly negatively correlated [88]. The inflammatory cytokines produced in visceral fat elevate serum hs-CRP levels, which are positively correlated with some metabolic alterations. The mean hs-CRP level rises significantly in obese versus normal-weight children and is positively correlated with BMI. However, the increase in hs-CRP levels was lower than that during acute inflammatory or infectious episodes and significantly increased in cases of severe (morbid) obesity [93]. Therefore, VDD may contribute to IR and the development of MetS secondary to inflammation.
The relationship between hemoglobin A1c (HbA1c) and 25(OH)D concentrations was independent of BMI, further establishing an independent association between vitamin D status and glucose homeostasis in obese adolescents [71]. Galunska et al. [93] conducted a pilot study examining the relationship between vitamin D status and MetS biomarkers in prepubertal Bulgarian children and adolescents. They found that more than half (57.1%) of the children were vitamin D-deficient, with a high prevalence in girls. A trend was noted in a negative correlation between 25(OH)D and fasting insulin, HOMA-IR, and WC. A noteworthy inverse relationship was observed between the PTH and 25(OH)D levels of the entire cohort. In this study, despite the non-significant difference between the obese and nonobese groups, the increase in hs-CRP levels in the obese group was closer to the upper reference limit. Although not significant, the reduced calcium and 25(OH) D levels in the obese group significantly increased intact PTH (iPTH) levels [93].
In a cross-sectional study of Polish children and adolescents, a decrease in serum 25(OH)D concentration by 1 ng/mL (2.5 nmol/L) was associated with increased fasting glucose by 0.238 mg/dL (0.013 mmol/L), TC by almost 1 mg/dL, and HDL-C by 0.565 mg/dL [91]. Censani et al. [95] observed a negative association between low serum 25(OH)D (<20 ng/mL) and non-HDL-C levels among overweight/obese children and adolescents, suggesting a negative effect of VDD on lipid markers with an increase in cardiovascular disease risk among children and adolescents with low vitamin D levels [95]. Vitamin D levels were positively associated with high HDL-C levels [65,91,92,116,117], indicating the role of vitamin D in the evolution of MetS and cardiovascular disease since VDD predisposes individuals to low protective HDL-C levels [65]. This is likely due to the role of vitamin D in maintaining adequate concentrations of apolipoprotein A-1, the main component of HDL-C [65,87]. During puberty, increased insulin levels, IR, and TG levels and decreased insulin sensitivity were significantly associated with reduced 25(OH)D levels independent of sex, BMI, and pubertal stage. Additionally, non–insulin-resistant overweight or obese children who developed IR during puberty showed a significant decrease in 25(OH) D concentrations over time accompanied by reduced HDL-C concentrations and increased TG levels and WC [68].
In a recent cross-sectional study in China of 6,091 children and adolescents, individuals of both sexes with VDD were at significantly increased risk of hyperglycemia, while girls were at risk of hypertension and high TG levels [15]. A clinical study in Italy reported that higher 25(OH)D levels were associated with a reduced incidence of MetS. A possible mechanism by which vitamin D may be associated with TG levels is through increased activity of lipoprotein lipase, which is regulated by vitamin D levels in adipocytes. Emerging evidence suggests that circulating 25(OH)D levels decrease significantly during the acute-phase response, providing another mechanism by which lower 25(OH)D levels may be a response to rather than antecedent of the disease [87]. Gul et al. [22] reported that children with hypertension exhibit lower 25(OH)D levels. Consistent with many recent studies, hypertension in obese patients is associated with reduced vitamin D levels. The antihypertensive properties of vitamin D include renoprotective effects, RAAS suppression, direct effects on vascular cells, and effects on calcium metabolism [15,22,87]. Through its effects on vascular smooth muscle, VDD alters vascular homeostasis and decreased arterial compliance, increasing the risk of atherosclerosis. Carotid intima-media thickness (cIMT) is considered a surrogate marker and subclinical indicator of atherosclerosis; each one-ln-unit increase in iPTH and 25(OH)D/iPTH ratio was significantly associated with a 53% and 39% increased risk of a high cIMT, respectively, among obese men in a cross-sectional study in Iran [118].
In a cross-sectional study of 90 newly diagnosed patients with Hashimoto’s thyroiditis (HT), a lower mean 25(OH)D level was observed in patients with HT versus healthy controls, while the prevalence of HT was 2.28 times higher in individuals with a 25(OH)D level <20 ng/mL. In the entire patient group, serum 25(OH)D levels were positively correlated with serum total thyroxine levels. Serum thyroid-stimulating hormone levels are significantly higher in patients with 25(OH)D deficiency versus insufficiency [119]. Epidemiological studies demonstrated that an individual's risk of developing type 1 diabetes mellitus (T1DM) is 8–15 times greater when a first- versus second-degree relative has diabetes [120]. Bae et al. observed that the mean serum vitamin D level was significantly lower and VDD prevalence was significantly higher in patients with T1DM versus healthy controls [121-123]. In this study, serum 25(OH)D levels in patients with T1DM were negatively correlated with age and PTH level and positively correlated with calcium and magnesium levels [121]. A recent study by Gülcü Taşkın et al. observed that median 25(OH)D levels were significantly decreased in older age groups: 53.8% of patients aged <5 years, 47% of patients aged 5–11 years, 38.5% of patients aged 11–14 years, and 16.2% of patients aged >14 years had 25(OH)D levels >30 ng/mL [124].
In a prospective cross-sectional study of 120 children and adolescents with T1DM aged 3–20 years, 37.5% had serum vitamin D levels <20 ng/mL, while 10.8% had secondary hyperparathyroidism. Although the mean PTH level in the vitamin D-insufficient group was below the cutoff value for hyperparathyroidism, it was significantly higher than that in the vitamin D-sufficient group [125]. No significant correlations between serum 25(OH)D and HbA1c levels [61,125,126], duration of diabetes mellitus (DM) [125,127], or metabolic parameters were identified [125]. In contrast, Bin-Abbas et al. [126] observed a negative correlation between 25(OH)D level and DM duration. In addition, the prevalence of secondary hyperparathyroidism was low in patients with VDD [126,127]. The PTH levels never fell to <20 pg/mL, which is clearly above the level of 10 pg/mL expected for continuous tonic secretion of PTH, suggesting that continuous tonic PTH secretion might be elevated in subjects with DM. Hypocalcemia may be too mild to induce an increase in PTH levels [127].
In a retrospective cross-sectional study of 301 children and adolescents aged 1–18 years with known T1DM, serum vitamin D levels were inversely associated with the frequency of hypoglycemia, BMI, diastolic blood pressure, and TG levels in males but inversely associated with current age, age at diagnosis, and TG levels in females. However, no significant correlation between HbA1c and VDD was observed, and only a small proportion (26.2%) of the patients had satisfactory HbA1c levels [128]. In a case-control study of 132 children, blood glucose, HbA1c, and inorganic phosphorus levels were significantly higher in patients with T1DM, whereas the serum levels of calcium and 25(OH)D were significantly lower. In this study, 84.8% of patients had 25(OH)D levels < 25 ng/mL [129].
In a case-control study conducted in Cairo, Egypt, of 50 patients with T1DM and 50 healthy children and adolescents, a statistically significant negative correlation was observed between HbA1c level and vitamin D status. Vitamin D levels were significantly lower in newly diagnosed patients and those with a disease duration of <5 years [130]. In Iraq, 51 children aged 2–12 years were recruited to analyze the association between immunological markers IL-10 and IL-17 and vitamin D insufficiency in children with T1DM. In this study, the mean HbA1c level of patients with T1DM was significantly higher than that of the control group, despite receiving insulin therapy. Additionally, the mean serum vitamin D and IL-10 levels were significantly lower in patients with T1DM versus controls. However, the serum IL-17 levels were higher in patients with T1DM versus control group. The ability of IL-10 to reduce inflammation has been established, suggesting that it may be useful for preventing the development of DM [131].
In a cross-sectional study of patients aged 5–18 years, the expression of FOXP3, a key gene in the development of Treg cells, and serum vitamin D levels were lower among patients with newly diagnosed chronic autoimmune thyroiditis (CAT), although Treg cells did not differ from controls. A 2- to 5-month vitamin D intervention increased FOXP3 expression and decreased thyroid antibodies, whereas Tregs remained the same in patients with CAT. Vitamin D intervention induced FOXP3 expression in children with CAT [132].
Endocrine-related disorders and VDR gene polymorphisms
Several studies have shown contradictory findings on the polymorphisms of VDR gene and development of vitamin D endocrine-related diseases. Relevant studies were presented as summaries in Table 3. d’Alésio et al. [55] hypothesized that SNPs located in the promoter region of VDR might influence VDR expression and, therefore, be more tightly associated with phenotypic traits or diseases. In a study conducted among 185 Caucasian female adolescents, the activity of the human VDR gene promoter (1a promoter), the SNP 1521G/1012A haplotype, was nearly twofold higher than that of the 1521C/1012G haplotype, suggesting that VDR content is reduced in cells (and individuals) bearing the CC/GG genotype. Of particular interest, the height of CC/GG girls was markedly shorter during the 4-year survey that spanned the pubertal growth spurt to adult height [55]. Interestingly, hVDRp genotypes were associated with height but not body weight, BMI, bone mineral content, or circulating markers of bone metabolism (osteocalcin and alkaline phosphatase activity). Thus, the effect of the putative hVDRp genotype on growth might specifically concern growth plate cartilage function rather than bone growth and mineralization [55].
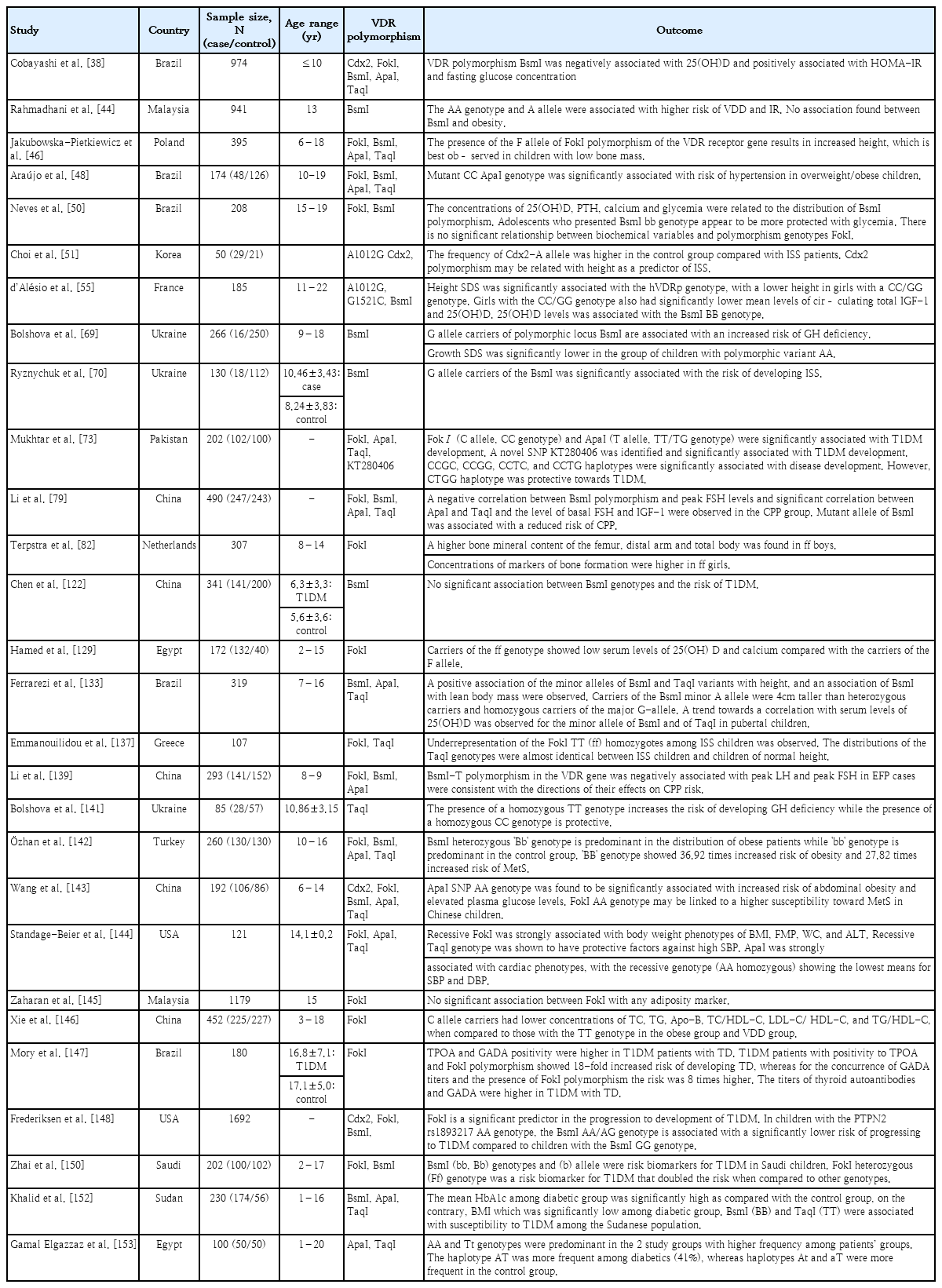
Vitamin D receptor gene polymorphisms and their association with vitamin D endocrine-related diseases in children and adolescents
Jakubowska-Pietkiewicz et al. [46] showed an association between FokI polymorphisms and increased body height in children and adolescents with low bone mass irrespective of sex and age. In a previous study by Ferrarezi et al. [133], minor BsmI and TaqI were positively associated with increased height, with carriers of the minor BsmI A allele being 4 cm taller than heterozygous and homozygous carriers of the major G allele. BsmI was independently and positively associated with body height and explained 46% of the variance in this trait. In a cross-sectional retrospective study of 72 children of varying pubertal status, a significant association between the FokI polymorphism of the VDR translation initiation site, total calcium absorption, and whole-body bone mineral density (BMD) was observed. The FokI gene is associated with variations in calcium absorption during early puberty as well as total bone mass and bone mass accretion during puberty. The effect of the homozygous ff genotype is substantial because approximately 20% less calcium is absorbed and accreted into the bone during pubertal growth, making the FF genotype a favorable polymorphism for enhancing calcium absorption [134]. The significant effect of the FokI polymorphism on height, bone formation, and bone resorption seems primarily related to its effect on calcium absorption [134]. In a study among European-Caucasian females, no association was observed between FokI polymorphisms and BMD, although there was a trend toward lower lumbar spine BMD (z score) in ff versus FF prepubertal girls [135].
In a study of 114 Australian children, girls with the TaqI TT genotype were approximately 4 kg heavier and 4 cm taller than those with the tt genotype [136]. VDR FokI and TaqI polymorphisms were genotyped in a group of children with idiopathic short stature (ISS) and healthy children from northern Greece. In this study, an association between FokI and ISS was observed, with the minor FokI TT genotype being underrepresented among children with ISS versus controls [137]. A genome-wide linkage study of 92 German families, each with 2 children with ISS, including constitutional delay of growth and puberty and familial short stature, provided significant evidence for the linkage of ISS in children to chromosome 12q11 and the association of VDR FokI with stature. At the population level, this variant might be responsible for ~34% of ISS cases, with the hetero- and homozygous presence of the risk allele, leading to an estimated population-attributable risk of 34%. This effect was similar in males and females, with a transmission ratio of 58% for the FokI-G allele in both sexes. However, both VDR A1012G (62%) and G1521C (61%) showed overtransmission in affected females only, which might explain the observation of a stronger linkage in sister-sister pairs [138]. In Korea, the frequency of the Cdx2 AA genotype decreased to 6.9% in patients with ISS, suggesting an association between Cdx2 and height as a predictor of ISS [51].
The ApaI polymorphism is associated with menarche at an earlier age in South Korea [84]. A genetic association study of 243 healthy control females and 247 females with CPP conducted in China observed an association between VDR TaqI, ApaI, BsmI, IGF-1, and FSH levels in the CPP group [79]. The negative correlation between the VDR BsmI polymorphisms and peak FSH levels suggests a protective effect against CPP risk by affecting GnRH-stimulated peak FSH levels [79]. Similarly, the BsmI-T allele is negatively associated with peak LH and FSH levels in Chinese girls with early fast puberty [139]. Moreover, a significant negative correlation between ApaI and TaqI and basal FSH and IGF-1 levels was observed in the CPP group. This suggests that VDR TaqI polymorphisms modulate IGF binding protein-3 mRNA expression, reducing IGF-1 levels, and therefore exhibiting a protective effect against earlier pubertal onset [79]. Ryznychuk et al. [70] conducted a study of 130 Ukrainian children and adolescents to investigate the clinical and genetic characteristics of children with ISS. In the present study, G allele carriers of the BsmI polymorphism were significantly associated with an increased risk of developing ISS. In the present study, IGF-1 levels were within the normal range; however, the lowest rate was observed in patients with the polymorphic variant GG VDR BsmI [70]. In a study conducted in China of prepubertal children with ISS and healthy age and sex-matched children, individuals with the Cdx2 AG genotype in the ISS group showed significantly higher growth rates than those with the GG genotype. However, polymorphisms in the VDR promoter may not be responsible for the ISS pathogenesis in Chinese children [140].
Bolshova et al. [69] observed an association between G allele carriers of the BsmI polymorphic locus and an increased risk of growth hormone deficiency (GHD). The risk of GHD increased in the presence of the BsmI GA genotype, whereas the risk of GHD was minimal in the AA genotype. Vitamin D levels were significantly lower in children with polymorphic variant G/C than in those with other VDR BsmI polymorphisms. It is likely that 1,25(OH)2D acts on human pituitary VDR, stimulating GH secretion and modulating gene expression [69]. Bolshova et al. [141] investigated the association between TaqI polymorphisms and GHD. In the present study, the proportion of the TC genotype was 1.28 times higher in children with GHD. The presence of the homozygous TT genotype increased the risk of developing GHD. Carriers of the T allele of the polymorphic locus TaqI (TaqI) of the VDR are associated with an increased risk of GHD.
Among Turkish adolescents, those with the BB and Bb genotypes of VDR BsmI were at an increased risk of obesity, MetS, and hepatic steatosis compared to those with the bb genotype. The BsmI heterozygous Bb genotype was predominant in the obese patients, whereas the bb genotype was predominant in the control group. The BB genotype showed 36.92 times increased risk of obesity and 27.82 times increased risk of MetS [142]. In Malaysia, BsmI showed no significant differences across genotypes for obesity parameters in adolescents aged 13 years. However, in the present study, the BsmI AA genotype was associated with a higher risk of VDD and IR [44]. Similarly, in a cross-sectional study of 208 Brazilian adolescents, those with the BsmI bb genotype appeared to be more protected against hyperglycemia. Adolescents with the BB genotype had a 115% risk of above-median glycemia levels; when the genotypes were analyzed together with BB + Bb, the risk was 89%, demonstrating that the presence of the B allele may increase the likelihood of higher glycemia levels [50].
Wang et al. [143] recently revealed a positive association between ApaI and overweight/obesity as well as glucose and TG levels. In addition, the FokI AA genotype was significantly associated with an increased risk of MetS, suggesting that VDR polymorphisms play a role in MetS development in children. In 2 cohort studies of Hispanic participants, FokI GG genotype carriers had the lowest mean BMI, FMP, hip and WC, and alanine aminotransferase levels, suggesting a protective effect on obesity phenotypes and measures of liver function [144]. In contrast, Zaharan et al. [145] found no association between adiposity phenotypes and FokI polymorphisms in Malaysian adolescents. Araújo et al. [48] demonstrated an association between the ApaI VDR variant and an almost sixfold increase in the risk of hypertension in Brazilian children and adolescents. In 2 cohort studies of Hispanic participants, the VDR TaqI and ApaI polymorphisms exerted protective effects on blood pressure among those aged <18 years. In the patients <18 years old, the TaqI-dominant genotype (AA homozygous) showed the highest mean systolic blood pressure (SBP) of 113.1 mmHg, whereas both the heterozygous genotype (AG heterozygous) and recessive genotype (GG homozygous) had lower mean SBP values of 106.8 mmHg and 95.3 mmHg, respectively. All genotype means were within the normal range for blood pressure; however, the recessive TaqI genotype was protective [144]. In a cross-sectional study of 452 Chinese children and adolescents, FokI C allele carriers with VDD had lower levels of TC, TG, apolipoprotein B, TC/HDL-C, LDL-C/HDL-C, and TG/HDL-C than obese children and adolescents with the TT genotype. Among those with low vitamin D levels, obese children with the FokI C allele may be at lower risk of cardiovascular disease than those with the TT allele [146].
In an evaluation of VDR FokI, thyroid dysfunction, and thyroid autoimmunity among Brazilian patients with T1DM, FokI polymorphisms were associated with the persistence of thyroid dysfunction, anti-glutamic acid decarboxylase autoantibody (GADA), and thyroperoxidase autoantibody (TPOA) positivity. TPOA and GADA positivity rates were higher in patients with T1DM and thyroid dysfunction than in those without thyroid dysfunction. Patients with T1DM with TPOA positivity and FokI polymorphisms showed an 18-fold increased risk of developing thyroid dysfunction, whereas those with concurrent GADA titers and the FokI polymorphism were at an eightfold higher risk [147]. The VDR FokI GG genotype was associated with progression to T1DM in islet autoimmunity–positive children, while VDR BsmI AA/AG significantly interacted with PTPN2 rs1893217 AA to increase the risk of progression to T1DM [148].
A study group consisting of 200 trios (2 parents and their child diagnosed with T1DM before 15 years of age) revealed that the TT genotype at SNP ApaI had a protective effect against T1DM. The BsmI-ApaI-TaqI haplotypes, A-G-T and G-T-C, were under-transmitted from parents to affected offspring and related to T1DM [74]. In 160 case–parent trio samples from southern Croatia, overtransmission of the Tru9I allele G and under-transmission of the Tru9I-BsmI A-A haplotype from parents to affected children were observed [149].
In a recent systematic review and meta-analysis of 40 case-control association studies, a subgroup analysis indicated significant associations between FokI and BsmI polymorphisms and T1DM risk in African and American populations [150]. In a cross-sectional study of 100 Saudi children with T1DM, the BsmI b allele was a risk biomarker for T1DM, whereas children with the FokI heterozygous genotype were at twofold risk for T1DM. In contrast, the FokI ff and f alleles appeared to be responsible for preventing T1DM [151]. A study conducted among Sudanese children and adolescents observed a higher frequency of the TaqI TT genotype and T allele and BsmI BB genotype and B allele among patients with T1DM and poor metabolic control compared to a good metabolic control group [152]. Mukhtar et al. [73] observed a significant association between FokI, ApaI, and the newly identified SNP KT280406 in Pakistani children and adolescents with T1DM. In the genetic analysis, “C” was identified as a risk allele from FokI. The TaqI-FokI-ApaI-KT280406, CCGC, CCGG, CCTC, and CCTG haplotypes were significantly associated with T1DM development, whereas the CTGG haplotype was protective against T1DM [73]. In a study Egypt of 50 children and adolescents diagnosed with T1DM and their healthy counterparts, a genetic analysis revealed that the AA genotype of ApaI and the Tt genotype of TaqI were predominant in the patient group, whereas the aa and tt genotypes were predominant in the controls, which may explain the possible protective effect of these genotypes against T1DM. The combined AATt, AAtt, and aaTT genotypes offered protection against T1DM, as they were more frequently noted in the control group [153]. However, in a recent study, children with T1DM more commonly had the ApaI and BsmI b alleles [154]. In contrast, a case-control study of 141 patients with T1DM in China did not observe any association between BsmI polymorphisms and the risk of T1DM [122]. Similarly, in a case-control study of 172 Egyptian children, FokI polymorphisms had no significant role in T1DM susceptibility [129].
Conclusion
The prevalence of VDD among children and adolescents has increased regardless of geographical location, affecting more females than males. In addition to socioeconomic factors and a sedentary lifestyle, the prevalence of VDD is most likely caused by environmental and religious factors. In addition to bone metabolism, the role of vitamin D as a hormone in glucose homeostasis, lipolysis regulation, and immune cell proliferation emphasizes its importance in the pathogenesis of endocrine diseases. Several studies have identified an association between vitamin D and endocrine-related disorders due to the effect of a single point mutation in the VDR gene. However, the effect of VDR polymorphisms on diseases is lacking consensus among researchers and often contradictory. This review revealed differing effects of VDR polymorphisms among and even within a certain population, most likely due to the ethnicity-dependent distribution of the VDR allele. Nevertheless, the effect of the VDR gene on the risk of disease progression should not be dismissed. Thus, ethnic- and population-based studies should be performed to better understand the effects of VDR gene polymorphisms on endocrine-related disease progression.
Notes
Conflicts of interest
No potential conflict of interest rele vant to this article was reported.
Funding
Dana Universiti Teknologi MARA Selangor Branch (DUCS), grant numbers 600UiTMSEL (PI.5/4)(161/2022).
Author contribution
Conceptualization: NFHR, NSMN; Data curation: NFHR; Formal analysis: NFHR; Funding acquisition: RAA; Methodology: NFHR; Project adminis tration: NFHR, NSMN, RAA, SHSAK; Visualization: NFHR, NSMN; Writingoriginal draft: NFHR; KD Riew; Writing review & editing: NFHR, NSMN, RAA, SHSAK, KD Riew
Acknowledgements
The authors acknowledge institu tional support provided by Universiti Teknologi MARA (UiTM) (Dana Universiti Teknologi MARA Selangor Branch (DUCS), grant number 600UiTMSEL(PI.5/4)(161/ 2022)).